Welcome to the 1,097 newly Not Boring people who have joined us over the past two weeks! If you haven’t subscribed, join 203,901 smart, curious folks by subscribing here:
Hi friends 👋,
Happy Monday! Grab a coffee, shake off the weekend, and let’s talk fusion.
Energy is upstream of everything we write about here at Not Boring. Cheap, abundant energy means economic prosperity, sci-fi brought to life, and even, one day, flying cars. So when Helion announced that it planned to deliver commercial fusion power in 2028, our ears perked up.
Luckily, we’d been working on a fusion piece. Rahul Rana (who graduated from Rutgers last weekend – congrats Rahul!!) is both a student of the history of moonshot projects in the United States and smart enough to understand and break down everything going on in the world of fusion.
So we decided to team up on a piece explaining how fusion works, where we are in our progress towards commercial-scale fusion, and why the transition from government-funded to private sector should spell acceleration.
We are certainly not experts on fusion. Treat this as a ride-along as we learn about the science and history of fusion, where it stands today, and where we might be headed in the coming years, complete with an overview of some of the most promising fusion startups. It’s a topic we expect to hear a lot more about in the coming years.
Whether 2028 is realistic or not, the Fusion Race is on. This piece is meant to be your starting point on one of the next decade’s most important adventures.
Let’s get to it.
Today’s Not Boring is brought to you by… Wander
Wander is one of our most popular sponsors ever…and there’s good reason: the vacation homes are dope. If you’re looking to book a last minute MDW trip or a relaxing getaway this summer, you should check out Wander’s listings. Plus, Wander REIT presents a unique investment opportunity. The REIT just released its Q1 results, and it delivered as promised: 5.1% appreciation and 8% annualized income. As a Wander REIT investor myself, I just received my first dividend!
Wander is a network of smart vacation homes that come with inspiring views, modern workstations, restful beds, hotel-grade cleaning and 24/7 concierge service –– combining the quality of a luxury hotel with the space and comfort of a vacation home, only better. All of the Wander homes look like they are straight out of one of those luxury car commercials. As a Not Boring reader, you can use promo code notboring for $300 off your next trip. (offer expires Monday 6/5 at 11:59pm). Explore now.
But that’s only part of the story. Wander recently launched Wander REIT –– a first-of-its-kind vacation rental REIT that gives you the chance to own a piece of their magical portfolio of homes. With Wander REIT, accredited investors have the opportunity to earn income and diversify their portfolio without the headache and hassle of buying a property, renting it out, or maintaining it.
The Fusion Race
Packy McCormick x Rahul Rana
Two weeks ago, a startup funded and chaired by Sam Altman announced a big partnership with Microsoft. This time, instead of investing in Sam’s brain, Microsoft committed to buying his power.
Specifically, according to the press release:
Helion Energy (Helion) today announced an agreement to provide Microsoft electricity from its first fusion power plant. The plant is expected to be online by 2028 and will target power generation of 50 MW or greater after a 1-year ramp up period. The planned operational date for this first of its kind facility is significantly sooner than typical projections for deployment of commercial fusion power.
While Sam Altman selling to Microsoft invites obvious OpenAI comparisons, the better analogy may be to one of the companies founded by Sam’s erstwhile OpenAI co-founder: SpaceX.
Like SpaceX, Helion set an absurdly aggressive timeline in an industry not recently known for its speed. Like SpaceX, Helion is the beneficiary of billions of dollars and decades’ worth of government-funded research, of global competition and cooperation in the pursuit of civilization-scale goals.
And like SpaceX, Helion may mark a turning point in one of the coming decades’ most consequential industries, the baton handoff from the measured and fundamental world of government and academia into the chaos and haste of the market.
We’re using Helion liberally here. We don’t know who will win Fusion Race 2.0, and this isn’t a winner-take-all market. But Helion is a worthy stand-in for the growing number of privately funded fusion companies racing to bring the power of the stars to the earth’s electric grid, economically.
Imagine a bizarro relay marathon in which one runner carries the baton for the first 26.0 miles, opens up a backpack full of batons, and hands them out liberally to a waiting horde of sprinters to dash all-out for the final 0.2 miles. That’s the best analogy we can come up with for this moment in the Fusion Race.
Global governments are the marathon runner. From the race to develop thermonuclear weapons after World War II, to the $22 billion, 50+ year cooperative ITER reactor currently being built in France, to the National Ignition Facility’s ignition achievement in December 2022, governments have led fusion research efforts for the better part of eight decades.
The world’s governments have run a well-paced marathon, producing something akin to a Moore’s Law in fusion for half a century. The triple product – of density, temperature, and time – is the figure-of-merit in fusion, and it doubled every 1.8 years from the late 1950s through the early 2000s, as one type of reactor - tokamaks, a form of magnetic confinement fusion (MCF) - got larger and more powerful.
In December, scientists at the government-funded National Ignition Facility (NIF) at Lawrence Livermore made headlines when they achieved fusion ignition, or scientific energy breakeven, for the first time in a controlled environment That is, they got more energy out of the fusion reaction than they spent on the laser energy used to drive it. The NIF used a different approach: inertial confinement fusion (ICF).
The world rejoiced – fusion energy is possible! – and then sobered up – it was like a AA battery’s worth of energy and, experts pointed out, commercial-scale fusion power might not arrive for decades.
That’s the joke in fusion, that fusion power is “always 30 years away.” Funny joke, to be sure, but it may no longer be true.
We’re at the handoff point, from private to public, thanks to improving compute and machine learning, better magnets and materials, the diverse range of technical approaches that are finally becoming feasible, and dramatically increased capital availability for startups.
Companies like Helion, Commonwealth Fusion Systems, TAE Technologies, General Fusion, and Zap Energy are the sprinters. Armed with $5 billion in funding, most of which has come in just the past two years, and decades worth of research made feasible by new tools, fusion startups are locked in a mad dash to the finish in what might be called Fusion Race 2.0. At the end stands commercial fusion and a healthy chunk of the $15 trillion global energy market.
Magic can happen in this public-to-private handoff. After languishing for decades post-Moon Landing, SpaceX revitalized the space economy and enabled a constellation of new businesses driven by both a sense of adventure and a profit motive. The commercialization of space pulled in timelines for such sci-fi visions as asteroid mining, space manufacturing, space hotels, and even Mars habitation by decades.
We don’t pretend to know who the SpaceX of fusion will be – in fact, it may be a confluence of factors more than any one company that unlocks the fusion economy – but we suspect that we’re at a similar point in fusion’s evolution.
Commercial fusion may power (some of) our homes within the decade.
As humanity embarks on this leg of one of our most important journeys, we thought it would be useful to put together a little primer on what’s at stake, how fusion works, where it’s been, and where we’re heading. We’ll do that today by covering:
Why is Fusion Important?
How Fusion Works
A Brief History of Fusion
Plasma Progress
Fusion Race 2.0
The Fusion Startup Landscape
Energy Too Cheap to Meter, Too Soon to Believe
To start, let’s all get on the same page about why fusion is important in the first place.
Why is Fusion Important?
According to Greek mythology, Prometheus defied the gods by stealing fire and bestowing it upon humanity. Humans harnessed the power of fire to construct magnificent cities and propel the growth of their civilization.
It’s such an alluring myth that we still use it as a shorthand for human ambition, innovation, and the quest for knowledge in the face of danger to this day.
Fusion is the Promethean myth brought to life, minus the liver-eating Caucasian Eagle (we hope). It’s the engine that powers the stars. By wresting the secret of unlimited energy production from the heavens, humanity may once again construct wonders in the physical world and propel the growth of our civilization.
A few years ago, it might have been controversial to argue that the world should use more energy. The climate argument thrusted in the opposite direction – consume less energy and transition whatever energy we still need to use to clean, renewable sources like solar and wind. Degrowth is a losing argument, and thankfully, it is in the process of being replaced by a more optimistic one: the call for energy superabundance.
Instead of consuming less energy, this argument goes, we should produce unimaginable amounts of clean energy from all of the sources we can: solar, wind, geothermal, nuclear fission, and one day, fusion. With practically unlimited cheap energy, we’ll be able to suck CO2 out of the air, mitigate the effects of climate change, and have enough left over to meaningfully improve the quality of life for billions of people around the world. We like this argument.
Energy use is the bound of civilization. If you increase it, civilization grows and progresses. If you limit it, civilization stagnates. If you diminish it, civilization crumbles. Energy is the most fundamental determinant of a society’s success. It feeds us, houses us, wins wars, powers technology, and dictates policy. Even money is a mere proxy for energy.
More energy lifts all boats. Numerous studies have found a long-run, direct, causal, and bidirectional relationship between energy consumption and economic growth. Researchers found that a 1% increase in real GDP per capita increases total energy consumption by 0.6%. It works the other way, too—a 0.9% increase in electricity use in OECD countries led to a 1.7% increase in GDP. For non–OECD countries, a 2% annual increase in electricity use increases GDP by 3.8%.
Simply put, more energy means more progress, and vice versa, across the board. More energy, and the physical abundance it brings, would spur growth without accelerating inequality.
If you’re with us that more energy consumption is a good thing, then we have good news: humans are consuming more energy than ever before.
The challenge, of course, is that the sources of energy humanity has ridden to such great heights also happen to be bad for the environment. The CO2, methane, and other greenhouse gasses emitted when they’re burned pose an existential risk to the planet. Even if they didn’t harm the environment, fossil fuels will eventually run out, even if we find increasingly clever ways to dig them up.
So the goal is to not just replace fossil fuels with cleaner energy sources, but to produce oodles and oodles of clean, abundant energy, multiples more than we produce and consume today.
What would such a world look like?
Ben Reinhardt’s Making Energy Too Cheap to Meter is a great read if you want to get as fired up about energy superabundance as we are. In it, he argues that more energy both solves existing problems and unlocks currently-impossible possibilities.
Cheap, superabundant energy could make all of our environmental problems disappear. We could capture all excess CO2 in the atmosphere, desalinate and clean all water sources, achieve high enough temperatures to zap away waste, produce more fertilizer and food, and construct sustainable infrastructure.
Cheap, superabundant energy would uncap our ability to manipulate our physical environment. Reinhardt describes what such an “ergophilic” world might look like:
Sounds like sci-fi, but that doesn’t mean it won’t come true. In Where is My Flying Car?, J. Storrs Hall analyzed which old sci-fi predictions from the first half of the 20th Century came true, and which didn’t. The answer depended on those predictions’ energy intensity.
Innovations that don’t consume a lot of energy – those in the world of bits – mostly came true. Innovations that do require a lot of energy – those in the world of atoms – did not.
Thanks to the work of Reinhardt, J. Storrs Hall, Eli Dourado, Austin Vernon, and many more, this vision of an energy superabundant world is becoming more pervasive and desired. The question is: how do we make it happen?
Here at Not Boring, we’re maximalist minimalists in all things, including clean energy sources. We don’t think it’s solar vs. wind vs. nuclear vs. geothermal vs. fusion; we want to see more solar and wind and nuclear and geothermal and fusion and the batteries to store it and whatever else will get us more cheap, abundant, clean energy sooner.
Solar, wind, geothermal, and batteries aren’t particularly controversial. They’ve gotten a lot of good coverage and analysis. Casey Handmer’s piece on solar, We’re Going to Need a Lot of Solar Panels, is particularly excellent. Noah Smith’s The Decade of the Battery is great, too. Even nuclear fission is thankfully making a comeback, with 55% of Americans now supporting it. We recommend Julia DeWahl’s Nuclear Energy: past, present, and future and Johnny Harris & Cleo Abram’s WTF Happened to Nuclear Energy? to learn more:
But perhaps because fusion is so clearly good and “always 30 years away,” it’s not as well-understood. It gets put in the “yeah that will be awesome whenever we get it” bucket.
Two things, though:
We think that fusion power is actually closer than most people outside of the industry realize, and we’ll cover why below.
Fusion is fucking awesome.
There are a bunch of things that make fusion so appealing as an energy source:
Abundant Fuel Supply. The primary fuel for fusion, hydrogen isotopes like deuterium and tritium, are everywhere. Deuterium can be pulled out of seawater, for example, making it practically limitless.
High Energy Density. That’s compounded by the fact that fusion reactions are substantially more energy-dense than fossil fuels. A single gram of fusion fuel can generate as much energy as 10 metric tons of fossil fuel.
Environmentally Friendly. Fusion does not produce greenhouse gasses or long-lived radioactive waste, making it a cleaner alternative to fossil fuels and traditional nuclear fission. Nuclear waste concerns are overblown, but nothing beats something here.
Safety. Unlike nuclear fission, a fusion reaction is inherently safe because it requires precise conditions to occur. If those conditions are disrupted in any way, the reaction stops. (The flip side, of course, is that those conditions are really hard to achieve!)
Sustainability. Fusion reactions can self-sustain under the right conditions, once they’ve achieved ignition. The energy produced by fusion keeps the plasma hot enough to keep producing energy. This is how the sun will fuel itself for billions of years.
If we’re to live in a world of superabundant energy, fusion will undoubtedly play a role. If we’re to travel off the world and out of the solar system, fusion will undoubtedly play a role in that, too. Very few people disagree with those statements.
But will fusion arrive soon enough to make an impact on reversing climate change? Would more money, smart people, facilities, and approaches speed up progress to the point that it could?
We think so. The science is well-understood, it’s just taken a few decades for the technology and engineering to turn the science into reality.
How Fusion Works
There are very few things older than fusion.
Within about three minutes of the Big Bang, during Big Bang nucleosynthesis, the first fusion process in the universe occurred when protons and neutrons combined to form the nuclei of the lightest elements: hydrogen, helium, and lithium. It would take another 100 million to 200 million years for the universe to cool down enough for the first stars to form, and therefore for fusion as we know it to take place as hydrogen nuclei combined to form helium inside of stars.
Just 3.6 billion years later, humans began to figure out what was going on in there.
In 1905, Albert Einstein published his mass energy equivalence formula: e=mc2.
“The equation e=mc2, in which energy is equal to mass multiplied with the square of the velocity of light,” Einstein explained, “showed that a very small amount of mass may be converted into a very large amount of energy.”
This formula underpins both fusion and its mirror-image twin, fission. Because the speed of light squared is a very large number, a small amount of mass can unleash a tremendous amount of energy. Let’s use 1 kilogram as an example.
E = 1 kg * (3 x 108 m/s)2
E = 1 kg * 9 x 1016 m2/s2
E = 9 x 1016 joules
Unless you’ve taken physics recently, 9 x 1016 joules is probably meaningless to you, so we’ll put it in more approachable terms. New York City consumes roughly 60 billion kilowatt hours (kWh) of electricity per year. At 3.6 million joules/kWh, that means New York City uses around 216 x 1015 joules (216 petajoules) of electrical energy per year. So perfectly converted into electrical energy, 1 kilogram of mass could power New York City for roughly 5 months.
That is, the mass in a 1 liter bottle of water (which has a mass of 1 kg), could power NYC for five months.
The goal of both fission and fusion is to convert mass into energy as efficiently as possible, ideally achieving a self-sustaining reaction that requires minimal external energy.
In nuclear fission, a neutron strikes the nucleus of a heavy atom (typically uranium-235 or plutonium-239), which absorbs the neutron, becomes unstable, and splits into two or more lighter nuclei, releasing energy along with additional neutrons, which can then trigger more fission reactions in a chain reaction.
Fusion is almost a mirror image: in fusion, two light nuclei, typically the Hydrogen isotopes Deuterium and Tritium, merge to form a single heavier nucleus. The process releases energy because the total mass of the resulting single nucleus is less than the mass of the two original nuclei. The leftover mass becomes energy. e=mc2.
It looks simple, but the devil is in the details. Fusion needs a controlled environment of more than 100 million degrees Celsius and as dense as the core of the Sun for long enough for the reaction to ignite. The extreme heat strips away electrons from the atoms, creating a state of matter called plasma, which is confined and heated to the conditions necessary for the nuclei to overcome their natural repulsion and collide, leading to fusion.
It took physicists another 34 years after e=mc2 to figure that out, and to understand what exactly was happening inside the dense core of the stars.
Until 1939, physicists’ best understanding of what happens inside of stars was based on Lord Kelvin’s (yes, that Lord Kelvin) 19th century theory of gravitational contraction: stars are balls of gas, and as gravity pulls the gas in, it heats up, causing the star to emit light and heat.
In 1939, building on the previous year’s work by George Gamow and Edward Teller (who theorized partially correctly that stars produce energy through fusion as protons tunneled through the strong nuclear force that holds them together), two physicists, Hans Bethe and Carl Friedrich von Weizsäcker independently arrived at the proper explanation. They proposed that stars produce energy through a series of nuclear fusion reactions, known as the CNO cycle (carbon–nitrogen–oxygen), by which stars convert hydrogen to helium.
Coming as it did at the beginning of World War II, this theory was timely. In just 13 years, scientists had turned it into a working hydrogen bomb. Mercifully, by that time, in 1952, the War had ended and physicists turned their attention to more peaceful and productive uses for their new superpower.
A Brief History of Fusion
The bomb makers at Los Alamos figured out a clever trick: use fission to create fusion.
The Teller-Ulam configuration, named after the physicists who devised it, uses the energy from the fission bomb to compress and heat the fusion fuel to such an extent that the atomic nuclei begin to fuse together, releasing a huge amount of energy. The resulting hydrogen bomb, “Ivy Mike,” “yielded 10.4 megatons of energy – over 450 times the power of the bomb dropped onto Nagasaki – and obliterated Elugelab [the island on which the test was performed], leaving an underwater crater 6,240 ft. wide and 164 ft. deep where the island had once been.”
Of course, you don’t want your power plants to turn into mushroom clouds that leave craters in their place. But Ivy Mike was the first man-made fusion reaction and achieved a Q-factor – the ratio of energy released by the reaction to the energy required to trigger the reaction – significantly greater than 1. Nuclear fusion was possible, and governments around the world sprung into action to figure out how to harness it.
After World War II, in 1946, President Truman signed the Atomic Energy Act and established the United States Atomic Energy Commission as the successor to the Manhattan Engineer District, better known as the Manhattan Project.
Given the relative maturities of the two technologies, the AEC primarily focused on nuclear fission. But the Commission also laid the groundwork for research into nuclear fusion, spurred on by rumors true and false of progress abroad.
In 1951, Argentinian President Juan Péron announced that a scientist named Ronald Richter, who moved to the country from Germany after World War II, had achieved controlled nuclear fusion. The claim turned out to be false, but it accelerated the race worldwide.
Starting that same year, three separate projects pursuing three different approaches to plasma confinement sprung up and would form the basis for Project Sherwood, a classified US program in controlled nuclear fusion:
Stellarator: Designed by Lyman Spitzer at the Princeton Plasma Physics Laboratory, Stellarators are devices designed to confine hot plasma within magnetic fields in a twisted, torus-shaped configuration to sustain nuclear fusion reactions.
Toroidal Pinch: Designed by James Tuck at Los Alamos, toroidal pinch devices work by confining a high-temperature plasma a donut-shaped chamber using magnetic fields; the fields are generated in such a way that they 'pinch' the plasma, increasing its pressure and temperature to the point where nuclear fusion can occur. Tuck named the device the Perhapsatron, because perhaps it might be able to achieve a fusion reaction.
Magnetic Mirror: Designed by Richard Post at Livermore National Laboratory, magnetic mirror devices confine plasma within a chamber using magnetic fields that are stronger at the ends, reflecting the charged particles back to the center creating a "magnetic mirror."
Each of these designs faced their own unique set of challenges. Stellarators, while promising, were difficult to design and build due to their complex magnetic field configurations. Mirror machines suffered from issues with plasma confinement, where the plasma would escape from the ends of the device. Pinch devices had issues with instabilities in the plasma that disrupted confinement. While execution was a challenge in the 1950s, the ideas were ingenious: we’ll see many come back later.
In 1952, the UK began work on its own attempt, the ZETA (Zero Energy Thermonuclear Assembly) project, which attempted to use the z-pinch method. In this method, an electric current is shot through the plasma in a straight line along the z-axis, generating a magnetic field around it, which compresses the plasma.
ZETA is perhaps most famous for a series of "false positive" results. In 1958, the ZETA team announced that they had observed neutrons indicative of fusion reactions. However, subsequent analysis revealed that the neutrons were likely produced by instabilities in the plasma, not fusion reactions. Once again, a strong idea faced with the technological limitations of the era.
While western democracies were experimenting with a range of approaches, the reigning heavyweight champion would be developed a few years later on the other side of the world.
The photo above on the left captures Soviet Premier Nikita Khrushchev (the bald guy in the middle) visiting ZETA in 1956, a sign of the open international cooperation on fusion development. As it turned out, two years later, the Soviets would crack open the problem and reveal the approach that remains the leader to this day: the tokamak.
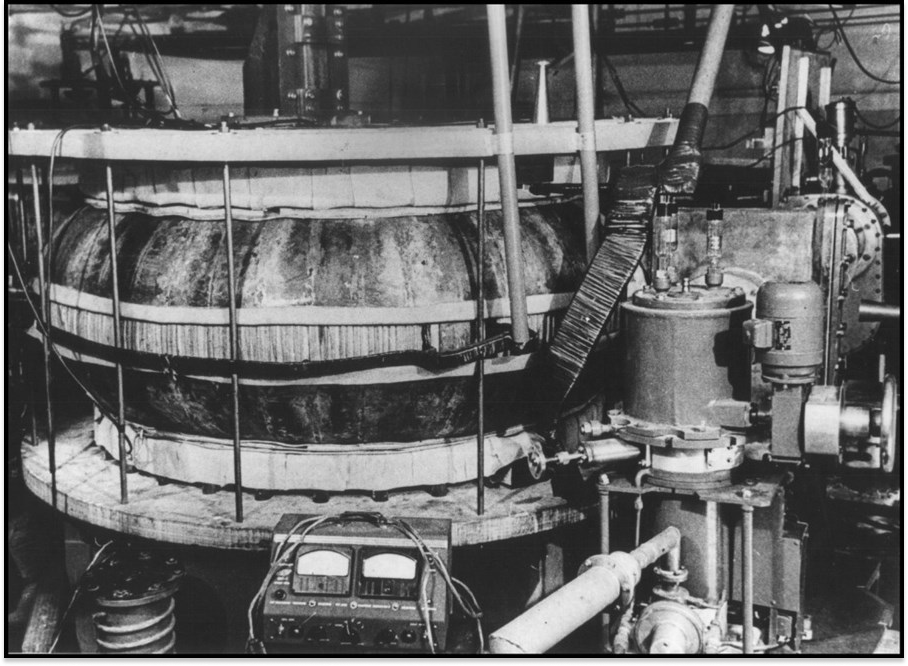
To the extent that you think about fusion reactors, the image that springs to your mind is probably one of a tokamak. The tokamak uses magnetic fields to confine a plasma in a doughnut-shaped configuration. This plasma is heated to extreme temperatures, causing atomic nuclei to collide and fuse together, releasing energy in the process. The magnetic field helps keep the hot plasma away from the walls of the machine, which is crucial for maintaining the conditions necessary for fusion to occur. Compared to early stellarator and pinch designs, tokamaks were more efficient, stable, and scalable.
The Soviets were on a roll. In 1957, they launched Sputnik. In 1958, they unveiled the tokamak. A decade later, in 1968, they announced that the T-3 Tokamak had achieved significantly higher plasma temperatures and densities than their western counterparts, around 1000 electronvolts (eV) or 10 million degrees Celsius.
The numbers beggared belief, so in a decision that would set the stage for ongoing international cooperation in fusion research, they invited a team of British physicists behind the Iron Curtain in 1969 to confirm the results, which they did.
That success led researchers around the world to converge on the tokamak design.
In the early 1970s, the conditions on the supply side (early tokamak progress) and demand side (high energy prices) formed the perfect storm for fusion excitement. The 1973 oil crisis sparked interest in sources of energy that would free the United States from its dependence on OPEC, and inspired a 6x annual government funding increase. Fusion was on a roll, too.
As the energy crisis abated, however, so did US funding for fusion research. In inflation-adjusted dollars, spending has leveled off at about one-third of its 1970s peak.
The parallels with space exploration are too on-the-nose to ignore. NASA’s budget shot up in the early 1960s to fund the Space Race, peaked at over 4% of the Federal budget in 1965 as Apollo spending ramped, and then quickly sinking to below 1% once the mission was accomplished, where it’s largely remained for the past 50 years. In 2020, NASA represented less than 0.5% of the Federal budget.
In the case of space, it would take 40 years for SpaceX to revitalize the industry.
In the case of fusion, while US funding slowed, fusion research became an international and increasingly cooperative affair, for better and worse.
Across the pond, the European Atomic Energy Community (Euratom) announced the Joint European Torus (JET) in 1977, began construction in 1978, and achieved plasma by 1983.
JET, a tokamak reactor, was the first to use tritium, produced 16 MW of fusion power from 24 MW of input heating power (Q=0.67) in 1997, and importantly, furthered confidence in the tokamak design, setting the stage for the largest tokamak ever built: the International Thermonuclear Experimental Reactor (ITER).
ITER was born a compromise.
In 1985, as the end of the Cold War approached, American President Ronald Raegan and Soviet Premier Mikhail Gorbachev convened in Geneva to establish a personal rapport and negotiate, among other things, a reduction in nuclear arms. While they couldn’t come to an arms control agreement during the summit, they did include as the last item on a joint statement a bullet that “emphasized the potential importance of of the work aimed at utilizing controlled thermonuclear fusion for peace purposes and, in the connection, advocated the widest practicable development of international cooperation in obtaining this source of energy, which is essentially inexhaustible, for the benefit of mankind.”
By the next year, in Reykjavik, the initiative was confirmed and a Quadripartite Initiative Committee was formed with Euratom and Japan. In 1987, in Vienna, the Quadripartite Committee agreed upon the name ITER. (More great history here.)
ITER, the largest tokamak reactor ever designed, has been the powerful magnet (pun intended!) at the center of fusion research since its inception, a collaboration among 35 countries (Russia, The United States, China, India, South Korea, Japan, and the European Union) across five decades. The project has five goals:
Achieve a deuterium-tritium plasma in which the fusion conditions are sustained mostly by internal fusion heating
Generate 500 MW of fusion power in its plasma on 50 MW of input (Q>10)
Contribute to the demonstration of the integrated operation of technologies for a fusion power plant
Test tritium breeding
Demonstrate the safety characteristics of a fusion device
The facility is still under construction in Saint-Paul-lès-Durance, France, and plans to generate its 500 MW of fusion power sometime between 2035 and 2040.
Achieving Q>10, whenever that happens, will be an incredible feat. At the same time, you have to wonder what the state of the fusion industry would be today if so many eggs hadn’t been placed in one huge, slow, expensive basket. The United States, one of the largest potential drivers of fusion progress, essentially outsourced much of its work to an international coalition, with all of the benefits and challenges that entails.
A 1976 report by the U.S. Energy and Research Development Administration, the R&D successor to the AEC (the regulatory arm was split off into the Nuclear Regulatory Commission (NRC)) proposed forty year funding scenarios, ranging from “Maximum effective effort” to “fusion never.” When Geoffrey Olynyk analyzed the actual fusion funding forty years in, he found that funding tracked below the “fusion never” scenario since the mid-1980s, right around the time the US decided to participate in ITER.
“Never fusion” turned out to be an overly dramatic description, and innovation continued, if slowly, through the 1980s through the 2010s. ITER has advanced the understanding of tokamak-based fusion, and a few promising non-tokamak approaches have been researched and tested in government-funded facilities through the period, most notably:
Stellarator. With modern technology to aid in its complex, twisted designs, the stellarator made a comeback. The world’s largest stellarator, the Wendelstein 7-X at the Max Planck Institute in Germany, began construction in 1995 and began operation in 2015. It was the first to be tested in silico before construction. Wendelstein’s goal is not to achieve energy production, but to test the long-pulse operation and efficiency of the stellarator design. It's designed to keep plasma in place for up to 30 minutes, which is important because a longer containment time increases the chances of fusion reactions occurring.
Field-Reversed Configuration (FRC) and Spheromaks. FRC and Spheromak are two plasma confinement designs in which plasma is held together by its own magnetic fields, reducing the need for complex external magnets. Both ideas were explored in the early days of fusion research and shelved, only to be revived over the past 20 or so years. There are no government-funded large scale FRC or Spheromak reactors, but as we’ll see, both are popular among startups.
Inertial Confinement Fusion (ICF). Most of the approaches we’ve discussed have been magnetic confinement fusion (MCF). Instead of magnets, ICF uses high-energy lasers or ion beams to rapidly heat the surface of a small pellet containing fusion fuel. Rapid heating causes the outer layer of the pellet to explode outward, producing a reaction force that compresses the remaining fuel inward. If the compression and temperature are high enough, fusion reactions occur in the compressed fuel, releasing energy.
Last December, scientists at the National Ignition Facility at Lawrence Livermore National Labs (approved in 1997 and completed in 2009) made ICF the most famous approach in the world when they used it to achieve ignition, or Q>1. Specifically, the NIF spent 2.05 MJ to generate 3.15 MJ. This is roughly a gain factor Q = 1.54 and is the first time in history that a gain factor Q ≥ 1 has been achieved in a controlled environment. Granted, the test has very little applicability to power plants, but it may be the Roger Bannister 4-minute mile moment for fusion, inspiring others by showing them it can be done.
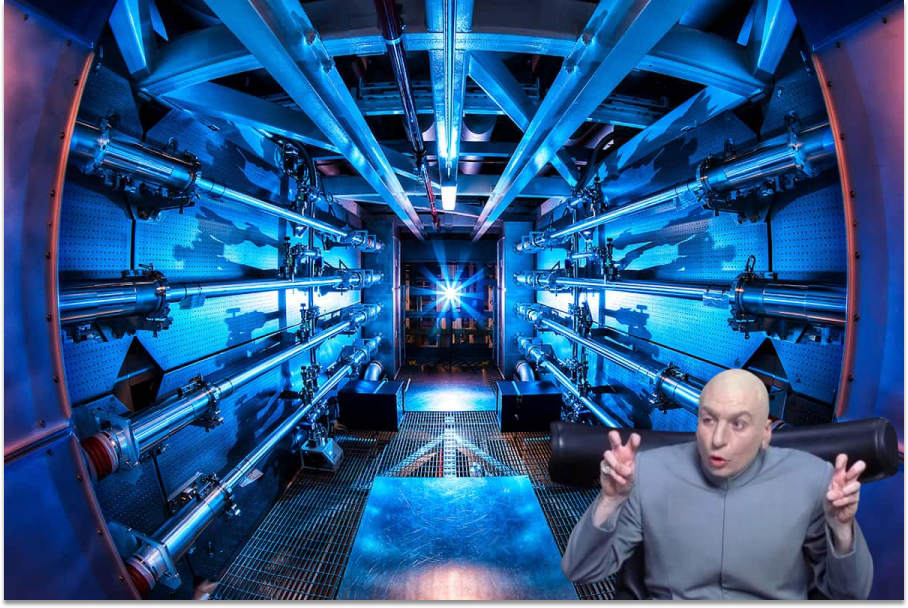
Until the 2010s, however, these novel approaches were few and far between (and relatively underfunded). ITER sucked most of the air out of the room.
Plasma Progress
To summarize everything in the last section, you can kind of picture fusion research over the forty years from the 1970s to 2010s as its own kind of fusion, many approaches compressed down to one main approach led by one international organization:
The period has been productive, though. Fusion rode its own kind of Moore’s Law from the T-3 through JET: from 1968 through 2000, the “triple product” doubled every 1.8 years.
The triple product is the figure-of-merit, the key metric, in fusion because it needs to reach a certain threshold for ignition, the point at which the fusion reaction becomes self-sustaining. It’s a product of density, temperature, and time:
n the density of ions in the plasma (ions/cubic meter)
T the temperature of those ions (keV2)
τE the energy confinement time (seconds)
Different types of systems attempt to achieve high triple products in different ways. Magnetic confinement fusion systems, like tokamaks and stellarators, strive for confinement times on the order of seconds to minutes at high temperatures but low densities. Inertial confinement fusion systems, like the laser-based system at the National Ignition Facility that achieved ignition (Q>1) in December 2022, have confinement times measured in nanoseconds, but make up for lost time, as it were, with higher densities.
The triple product is separate from the fusion power gain, Q, which measures the ratio of energy output to energy input. But they’re related: Q is proportional to the triple product.
To achieve a high Q-factor, you need to have a high triple product - that is, a plasma that is dense, hot, and well-confined for a sufficient amount of time. This would mean that the conditions are right for a lot of fusion reactions to occur, which would produce a large amount of energy. So the triple product is a good gauge of progress in fusion, and it’s been growing exponentially.
Or at least it had been, until it capped out with the Japanese tokamak JT-60’s record of 1.53×1021 keV·s·m−3, set in 2004.
Now, it seems, the fusion world is waiting for ITER to set a new record and achieve the Triple Product required for a D-T power plant some time between 2035 - 2040.
In a conversation with Lex Fridman, Dennis Whyte, former ITER scientist, Director of the MIT Plasma Science and Fusion Center, and inventor of the SPARC reactor used by Commonwealth Fusion Systems, explained ITER’s impact:
I worked most of my career on ITER, because when I came into the field in the early 1990s, you can’t imagine being more excited about something. ‘We’re going to change the world with this project.’ An entire generation just poured their imagination and creativity into making this thing work. Very good.
At some point, though, when it got to being another five years of delay, or a decade of delay, you start asking yourself, ‘Is this what I want to do?’...
I just want fusion energy, OK, cause I think it’s so important to the world. If that’s the case, then why do we have only one attempt at it on the entire planet, which was ITER. That makes no sense to me. We should have multiple attempts at this.
Whyte praises the contributions of ITER, and the importance of government-funded research to pushing the space forward, and he’s right. Pause to think about it. It’s near-miraculous that in less than a century since unraveling the stellar secret of near-limitless energy production, we stand on the cusp of harnessing that very power for our own use.
But he’s also right to say that we should have multiple attempts at this. With the entry of well-funded commercial players, the race to bring the power of the stars to the earth will only accelerate. We can’t afford to wait for ITER.
Fortunately, waiting a couple decades isn’t something that startups like to do. Like that bizarro marathon in which one runner runs the first 26 miles before handing off to waiting sprinters to run the final 0.2, a growing number of fusion startups are taking the baton from government-funded labs in an attempt to make commercial fusion a reality long before ITER ignites.
Fusion Race 2.0: Here Come the Startups
If government funded fusion efforts roughly look like this…
… then it’s heartening to see that the growth of private fusion companies looks like this:
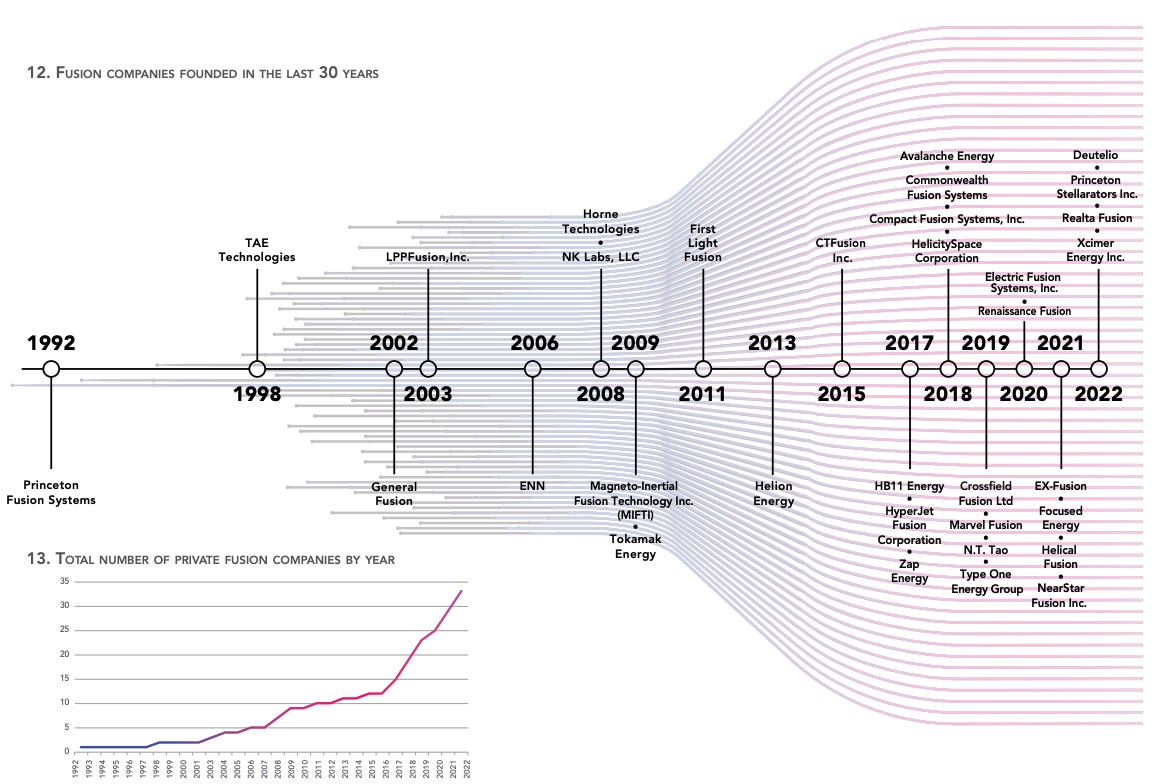
Much like the innovation in space launch transitioned from government-led to SpaceX-led, unleashing dramatically faster timelines, lower costs, and a growing ecosystem of space startups, fusion has become a viable commercial enterprise over the past decade, and especially over the past five years. This shift to free market experimentation typically pulls progress forward.
Why now? There are a bunch of reasons, some technical, some cultural.
Certainly, the $22 billion spent on ITER, and the billions spent here and there by world governments on a smattering of other approaches, laid the groundwork for the recent explosion.
The diaspora of talent from government-funded programs has also played a role. David Whyte left ITER and developed SPARC at MIT, and his students spun the technology out into Commonwealth Fusion Systems. Three of Type One Energy’s founders worked on the HSX Stellarator at the University of Wisconsin, and the fourth worked on Wendelstein 7-X. Former government and academic researchers sit atop and within many of today’s fusion startups.
But fusion researchers have worked in government-funded labs for decades. They’re able to jump into the commercial sector now because progress on a few different fronts has brought long-ago dismissed approaches back from the dead and made smaller-scale attempts feasible for the first time.
Compute. Modern fusion reactors rely on sophisticated computers that need to adjust in fractions of milliseconds to keep plasma stable. Just as your computer gets faster as Moore’s Law does its thing, so do reactors, which means more control with less hardware.
Machine Learning. Machine learning algorithms allow fusion researchers to run enormously complex simulations and access a much broader design space than before. As Lowercarbon explained, “Experiments that, just a few years ago, took years to physically design and setup, can now be executed with high-fidelity, entirely in the computer, in mere hours/days.” This introduces startup-like iteration speed to fusion, and makes complex old designs, like stellarators, possible.
Materials. Knowledge of materials science and additive manufacturing have vastly advanced, revolutionizing the processes for building lasers, electrodes, and, crucially, magnets. Most importantly, high-temperature superconductors (HTS) could mean smaller, more powerful magnets, enabling faster-to-market designs like Commonwealth Fusion Systems’ (CFS) SPARC reactor.
Taken together, these advances allow fusion to move at something closer to startup speed and scale than government speed and scale, with the risk-taking and fast iteration that entails. And funding mechanisms are catching up to that reality.
The US government has returned to funding diverse experiments in addition to its contributions to ITER. The White House recently put out a bold decadal plan for fusion commercialization and public-private partnerships alongside the IRA and the CHIPs Act. The establishment and empowerment of ARPA-E has transformed the government funding landscape for fusion from single, expensive bets to a more venture-capital style that spreads smaller bets across different organizations with different approaches to fusion.
Venture capitalists themselves have, of course, gotten in on the act, even raising dedicated fusion funds, implying that they believe fusion will be commercially ready within at most 10-15 years. Last year, Chris Sacca’s Lowercarbon Capital’s announced its Q>1 Fund, writing, “Fusion companies will be among the most valuable enterprises in history. More importantly, they will undoubtedly change more lives and give humanity a better shot than anything else that has come before.”
All told, scientific entrepreneurs have founded more than 50 fusion companies that have raised over $5 billion of private funding to date, plus an additional $117 million in grant money, much of it in the past two years. In 2021, just two companies – Commonwealth Fusion Systems ($1.8 billion) and Helion Energy ($500 million) – raised a combined $2.3 billion, with another $1.7 billion committed to Helion upon the completion of milestones. Several others, including TAE, Tokamak, General Fusion, and Zap Energy, have raised at least $200 million.
These numbers sound large, but combined, private fusion startups have raised less than a quarter of the $22 billion governments around the world have dedicated to ITER to date. If ITER’s goal is to crack fusion by going bigger, then collectively, these startups are competing with each other to do it better and faster.
The Fusion Startup Landscape
The leading fusion startups are diverse in their approaches and their geographies.
We’re tracking 33 fusion startups, thanks in large part to the great report put together by the Fusion Industry Association: The global fusion industry in 2022. There are likely more still in stealth. We put together the list in a handy Notion table: Fusion Startups.
For the more geospatially-inclined, we also put the startups, along with some of the big government-funded labs, in a Felt map: Fusion Startups and Labs.
(The IAEA maintains a comprehensive map, which we found after making ours: FusDIS)
Not all of these startups are created equal. Some people believe that CFS, Helion, and TAE are the only serious contenders at this point, and that all others are still science experiments. Funding is a useful but imperfect proxy for odds of success. That said, as fusion outsiders, we see the number of science experiments as a feature and a potential accelerant.
What’s most striking when looking at the list of fusion startups is that they’re taking such a wide range of approaches to fusion, a welcome departure from the ITER-only paradigm.
To be sure, a few are pursuing improvements on the tokamak design, but many more are using new technical capabilities to dust off old designs: stellarators, magnetic mirrors, field reversed configuration, z-pinch, spherical tokamaks, and laser-driven inertial confinement.
Others are taking approaches with names straight out of sci-fi: orbitrons, pulsed high density aneutronic fusion, plectonemic reconnection, plasma jet driven magneto inertial fusion, hypervelocity gradient field fusion, and muon-catalyzed fusion with high-density fuel.
Some wild and crazy scientists are even experimenting with fuels other than D-T (deuterium and tritium).
A few are using fuels such as proton-boron-11 (pB11) or Deuterium-Helium-3 (DHe3) to produce aneutronic fusion, a form of fusion that produces energy but does not produce neutrons, which can be beneficial because neutrons can be difficult to contain, damage reactors, and induce radioactivity in materials they collide with.
Perhaps more crucially from the perspective of commercialization, as Eli Dourado explained, aneutronic fusion may overcome traditional fusion’s inefficiency as a very “expensive way to produce steam” by directly converting the energy from plasma into electricity.
Different startups are trying different combinations of all of the above, mixing and matching approaches and fuels to see what works best. Helion and Princeton Fusion Systems are doing Field Reversed Configuration (FRC) with DHe3, TAE Technologies is doing FRC with pB11, and Compact Fusion Systems is kicking it old school with FRC using D-T.
Some fusion startups are going big, and others are going small. Germany’s Marvel Fusion has the biggest ambitions for its first plant – it has said that its first plant, which it plans to have live by 2027, could produce as much as 2,000 MWe, 400 times larger than Helion’s Microsoft plant. At the small end, Avalanche Energy plans to build Orbitrons that produce as little as 0.005 MWe (5 kWe) in a form factor the size of a lunch pail.
The wide range of power generation targets speak to the diversity of fusion startups’ target markets. Most of them have their eye on the big prize – electricity generation – but others, like Avalanche Energy and Electric Fusion Systems are looking to power planes, rocket ships, boats, trucks, cars, and small, portable transportation. (For a healthy dose of skepticism on these smaller systems – and a generally smart and balanced fusion conversation – check out the r/fusion subreddit.)
And perhaps most importantly, nearly all of them expect the first fusion power plant to deliver electricity to the grid before ITER comes online.
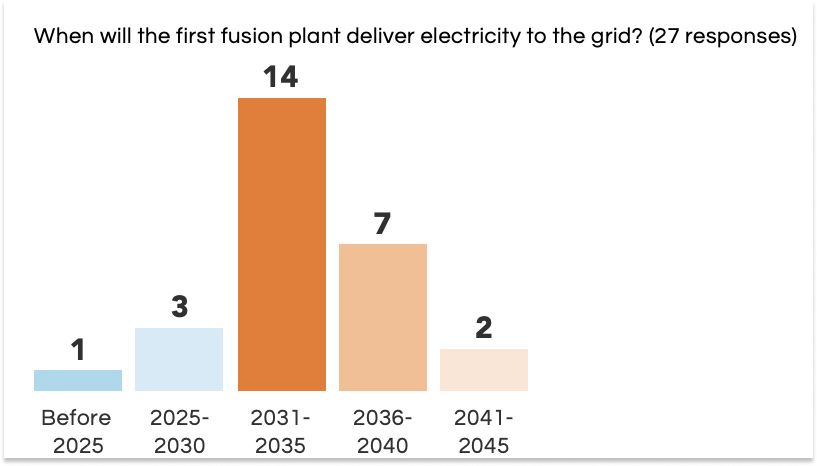
In fact, the vast majority of the startups on the list think that they’ll be able to beat ITER to market themselves. Only two of the thirty-three companies have a commercial target date in the 2040s, while seven fusion startups plan to sell fusion power in the 2020s!
Of course, the odds that any particular fusion startup hits its aggressive timeline are very small.
No company has ever produced commercial fusion power before. It’s insanely difficult, possibly the most difficult engineering challenge known to humankind. Most of the approaches they’re taking are wildly unproven. Plus, they’re incentivized to pronounce aggressive targets – with so much money flowing into the space, admitting that you won’t be able to produce fusion power is a good way to get ignored by VCs. The MIT Technology Review recently wrote a piece on experts’ doubt around Helion’s 2028 announcement, which is a good summary of the counter-arguments.
But we think the odds that none of them hit their target, or at least slip just a few years and still land in the early 2030s, are also small.
While ITER has been slowed down by cooperation, these startups are speeding up thanks to competition. While ITER may end up being a very expensive way to make steam, these startups will have to face market realities (aided by government-subsidies in the beginning) to produce power that is cheaper than the alternatives.
What’s notable about Helion’s recent announcement, then, is the combination of the 2028 timeline with its very difficult-to-achieve but more efficient aneutronic fusion approach. Speed and efficiency. It’s a nearly perfect encapsulation of how a market makes a company behave.
Now, the race is on. It remains to be seen which companies will be successful. Many of the startups on the list will undoubtedly fail, incinerating billions of dollars en route to a multi-trillion dollar prize. But a few will succeed, and when they do, fusion’s challenge – first from science to technology – will evolve once again - from technology to scale.
Energy Too Cheap to Meter, Too Soon to Believe
“It is not too much to expect that our children will enjoy in their homes electrical energy too cheap to meter, will know of great periodic regional famines in the world only as matters of history, will travel effortlessly over the seas and under them and through the air with a minimum of danger and at great speeds, and will experience a lifespan far longer than ours, as disease yields and man comes to understand what causes him to age. This is the forecast for an age of peace."
— Atomic Energy Commission Chairman Lewis Strauss in a 1954 address to scientists.
When AEC Chairman Lewis Strauss delivered his famous 1954 speech, he was confident that atomic energy – from both fission and fusion, or both – was just a generation away. He was off by a few generations. But his children’s children’s children’s children may well grow up in a world in which energy is indeed too cheap to meter.
This seems to be the way of things with any sufficiently important technology. Early participants set wildly ambitious timelines, which turn out to be off not by years but by decades. It’s the Gartner Hype Cycle at Decadal scale, and it happens over and over again. We wrote about it in Four Seasons Total Tech, highlighting five now-thriving industries that went through their own brutally cold winters.
When things begin to thaw, however, the ferocity with which they accelerate is hard to fathom and hard for even (or especially) the experts to appreciate. As one well-known example, solar industry experts consistently underestimate solar’s growth by hilarious margins.
The pattern has played out in AI, too. In 1957, Herbert Simon and Allen Newell predicted that within ten years, a computer would be the world’s chess champion, discover and prove a new mathematical theorem, write music “accepted by critics as possessing considerable aesthetic value,” and that most theories in psychology would take the form of computer programs.
Simon and Newell were off by about six decades, but we suspect that even they would be awed by the pace of progress in AI today.
Fusion may be approaching that same point in its eight decade history. From “always 30 years away” to “everything everywhere all at once.”
There are certainly still huge technical challenges to overcome. Like stars themselves, excitement around various approaches will heat up and burn out.
Once those are overcome, there will be the more mundane obstacles that face any scaling startup, particularly those that operate in the world of atoms. There will be permits to obtain and plants to build and construction delays and cost overruns. There will be quarterly earnings, and analysts asking questions of fusion CFOs about Q3 gross margin misses. One definition of commercial fusion is fusion that not only contributes a lot of power to the grid, but does so with predictable and scalable economics. There’s a lot of work to be done to get there.
There will undoubtedly be scandals, too, as the less scrupulous are attracted to the opportunity like plasma to magnets. There will be competition and bickering.
But all of those are incredible problems to have – they will mean that fusion power has become a real industry.
And we don’t think it’s hyperbolic to say that the fusion industry, when it becomes an industry, has a greater potential to change the world for the better than any in the history of humankind.
We’ll pull carbon out of the air, desalinate water, and incinerate waste. Cheap electricity will be a human right, and with it will come economic opportunity. We’ll be able to do luxuriously wasteful things, like keep the AC running with the windows open to let the fresh air in on a hot day, even in parts of the world that aren’t even able to run the AC with the windows closed today.
The second- and third-order effects are harder to predict, but even more fun to imagine. We can’t wait to scoot off to a last-minute dinner with friends in Tokyo, or to zip about in flying cars. More practically from our seat as investors, it’s exciting to think about the potential for atoms-based startups in a world of cheap, abundant energy. Geopolitically, what will we even go to war over when every nation has plenty of energy?
Whatever the specific impacts, energy too cheap to meter is a civilization-altering leap. The experts may be right, and we might still be decades away. But we believe there’s a better-than-commonly-accepted chance that by this time ten years from now, you’ll use devices powered by the same source of energy that powers the stars.
If nothing else, we hope this post makes you take that possibility seriously enough to do your own research and thinking, keep up with the r/fusion subreddit, follow the companies in our Fusion Startup Notion, and form your own hypotheses around how things will change if fusion comes sooner than expected.
We expect these companies to become household names in the coming years, and frankly, we can’t wait to live in a world that celebrates the people doing insanely hard things to give humankind an Archimedes Lever (while blasting AC in our hoverhomes).
Ad vim stellarum in terra.
Thanks to Rahul for the collaboration and to Dan for editing!
That’s all for today! We’ll be back in your inbox a couple times later this week 👀
Thanks for reading,
Packy
Can we chat?! My name's Ally and I'm Chief of Staff at CFS. We have been having many conversations lately about how to effectively help the world understand if a company, govt, or approach is on track. Drawing inspiration from the biotech world that has technical complexity and long time horizons to market but has well understood value inflection points along the way , we have been thinking about how to establish milestones for the fusion industry that are meaningful to all concepts, easy to understand, public, easy to measure. Especially since fusion we know is hard and it's hard to unpack. These milestones can help measure the race. Would love to brainstorm about it. Lmk and I'll send my email!
I'm curious if you came across a deeper dive on how big the impact of ML will be as an inflection point driver for speeding up fusion development. My own intuition is that it might well be the new tool needed to divine the correct settings needed to make it all work (particularly with Helion's approach), but I'm curious how that shiny tool obscures other technical obstacles. Any thoughts or references you might have stumbled across in your research would be super appreciated!